The same non-intuitive ideas can be found in complex systems and sometime mathematical models can help scientists to predict effects that you wouldn’t expect.
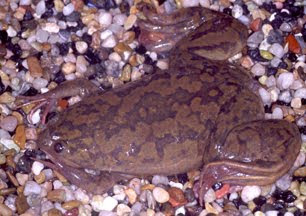
Claire Tomlin from UC Berkeley and Stanford University has been using models like these to understand responses to breast cancer treatment, using techniques developed on simple systems such as frog embryo development.
Professor Tomlin comes from a background of aeronautical engineering – in her book, if you have a system you should develop a mathematical model of that system, and then work from there. Taking the example of a plane in flight, you can add the inputs (thrust, elevation angle), measure the outputs (roll, forward velocity) and ensure a smooth flight by controlling the system through a feedback loop.
She showed us how the same types of differential equations she uses in engineering can be applied to biology, for example in the simple case of a species of frog embryo. This particular frog, Xenopus, has a pattern of hairs over its surface that helps it to trap nutrients as it develops. A hairy skin cell is always surrounded by smooth cells, and a smooth cell always has a hairy neighbour. Whether or not a cell is hairy is governed by two proteins – if the ‘hair’ protein is dominant in cell A, it suppresses the ‘smooth’ protein in that cell, giving you a hairy cell. In its neighbouring cell, the presence of ‘smooth’ promotes the influence of the ‘hair’ protein in cell A, giving you a feedback loop. Tinkering with the equations eventually gets you to the point where you can model reality accurately, and from there predict unexpected effects.
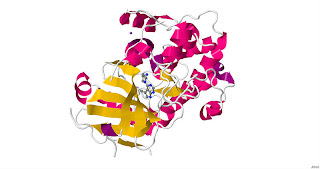
From here, Tomlin’s team has gone on to work on much more complex real life systems, including the HER2/HER3 protein pathway in breast cancer. HER2 is a protein found on the surface of certain cancer cells. Some breast cancers have a lot more HER2 receptors than others and the tumours are described as being HER2-positive. Tumours that are HER2-positive tend to grow more quickly than other types of breast cancer, often with a worse outcome for the patient.
High profile drugs have been developed to tackle these types of aggressive cancers, but they only work in about 60% of cases. In the other cases, the drug dramatically reduces the tumour’s growth for around 24 hours, but then the effect wears off and the growth continues more strongly than before. One particular drug, lapatinib, targets the protein AKt, which is involved in cell growth and cell death, known as apoptosis. By knocking back AKt levels in the tumour, this should increase the rate that the cancerous cells die and dramatically reduce the tumour’s growth – except 40% of the time, it doesn’t work, or not for long.
Tomlin’s team worked with the Karolinska Institute and others to model these complex pathways and found a surprising result – if you actually increase the levels of AKt in patients before you start the drug treatment, the outcome is dramatically better. This is a very counter-intuitive approach but the results seem to show that it works. In results only released a couple of weeks ago, cell death rates are improved from around 11% to 50% by boosting AKt levels at the start. Steering the system to a new dynamic equilibrium at the outset seems to do the trick, like flying a plane out of a turbulent patch. It’s early days, but Tomlin’s work could lead to a much better outcome for patients with hard to treat cancers.
No comments:
Post a Comment